A timecourse of ferroptosis observed by fluorescence live cell imaging using FerroOrange and ROSFluor series
Ferroptosis is a cell death which depends on intracellular labile Fe2+. Its mechanism is known as a distinct from that of apoptosis or necrosis. Excess amount of intracellular labile Fe2+ induces generation of reactive oxigen species (ROS) and lipid peroxidation, those lead to cell death. It has also been revealed that ferroptosis is induced in some neurodegerative diseases, and some tumor cells are ferroptosis resistant.
Here, we tried to detect labile Fe2+ that induces ferroptosis, as well as ROS, by using activatable fluorescent probes of FerroOrange, APF, OxiORANGEand HYDROP, in the timecourse of ferroptosis.
Visualization of Fe2+ and ROS in the process of ferroptosis
Erastin was applied to HT-1080 cells to induce ferroptosis, and intracellular labile Fe2+ and ROS were imaged after 3, 6, 9 hours after the application, using the fluorescent probes. FerroOrange (1 μM), APF (5 μM), HYDROP (1 μM), and OxiORANGE (1 μM) were applied 30 minutes before each of the observation timepoint. Fluorescence signal of FerroOrange which indicates labile Fe2+ was maximum at 3 hours after the induction, whereas fluorescence of other ROS probes: APF which detects hydroxyradical (·OH), hypochlorous acid (HClO), and peroxinitrite (ONOO–), OxiORANGE which detects hydroxyradical (·OH), hypochlorous acid (HClO), and HYDROP which specifically detects hydrogen peroxide H2O2 were maximum at 6 hours after the induction. These results indicate that in ferroptosis, ROS increase after the increase in labile Fe2+.
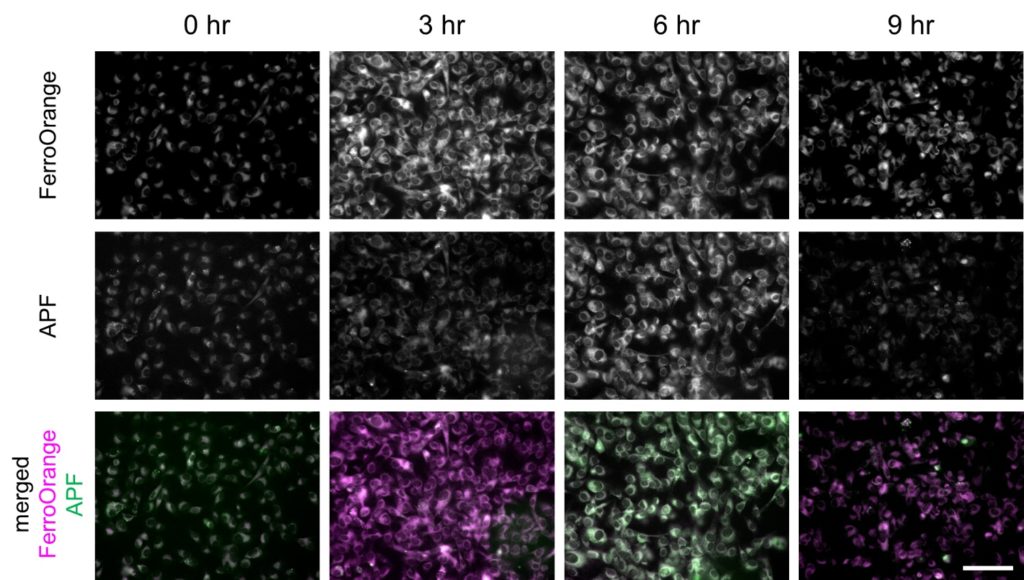
Fluorescence intensity changes of FerroOrange and APF in ferroptosis-induced HT-1080 cells
Fluorescence intensity of FerroOrange and APF in erastin-applied HT-1080 cells (upper and middle rows) were shown. Images were overlayed as pseudocolor images (bottom). Magenta indicates fluorescence of FerroOrange, green indicates that of APF. Bar indicates 100 µm.
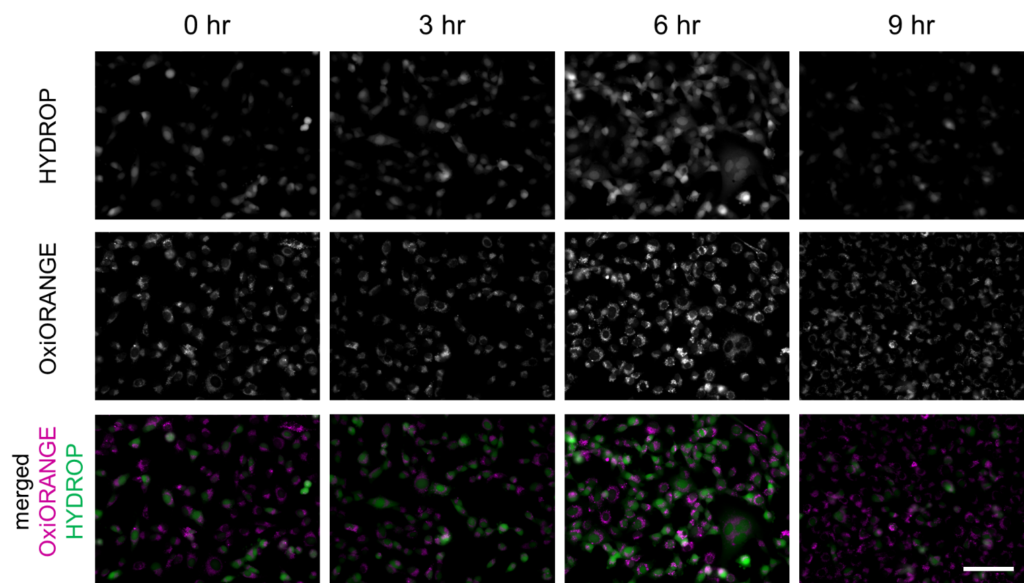
Fluorescence intensity changes of OxiORANGE and HYDROP in ferroptosis-induced HT-1080 cells
Fluorescence intensity of OxiOrange and HYDROP in erastin-applied HT-1080 cells (upper and middle rows) were shown. Images were overlayed as pseudocolor images (bottom). Magenta indicates fluorescence of OxiOrange, green indicates that of HYDROP. Bar indicates 100 µm.
Experiment procedure
- HT-1080 cells of 2 × 105 were seeded on glass bottom dishes and cultured until the cells attached to the bottom.
- Erastin (final concentration of 30 μM) was added to the media and cultured for2.5, 5.5, 8.5 hours at 37℃, 5% CO2.
- Each probes were added to the media and cultured for 30 minutes.
- The cells were rinsed twice with HBSS and observed by fluorescence microscopy.
※In different cell culture conditions, the concentrations of the reagents and incubation time might be varied. In this experiment, preliminary tests were needed to optimize those conditions.
※If the cell adhesion to the dishes was weak, we recommend to use poly-L-lysine coated dished.
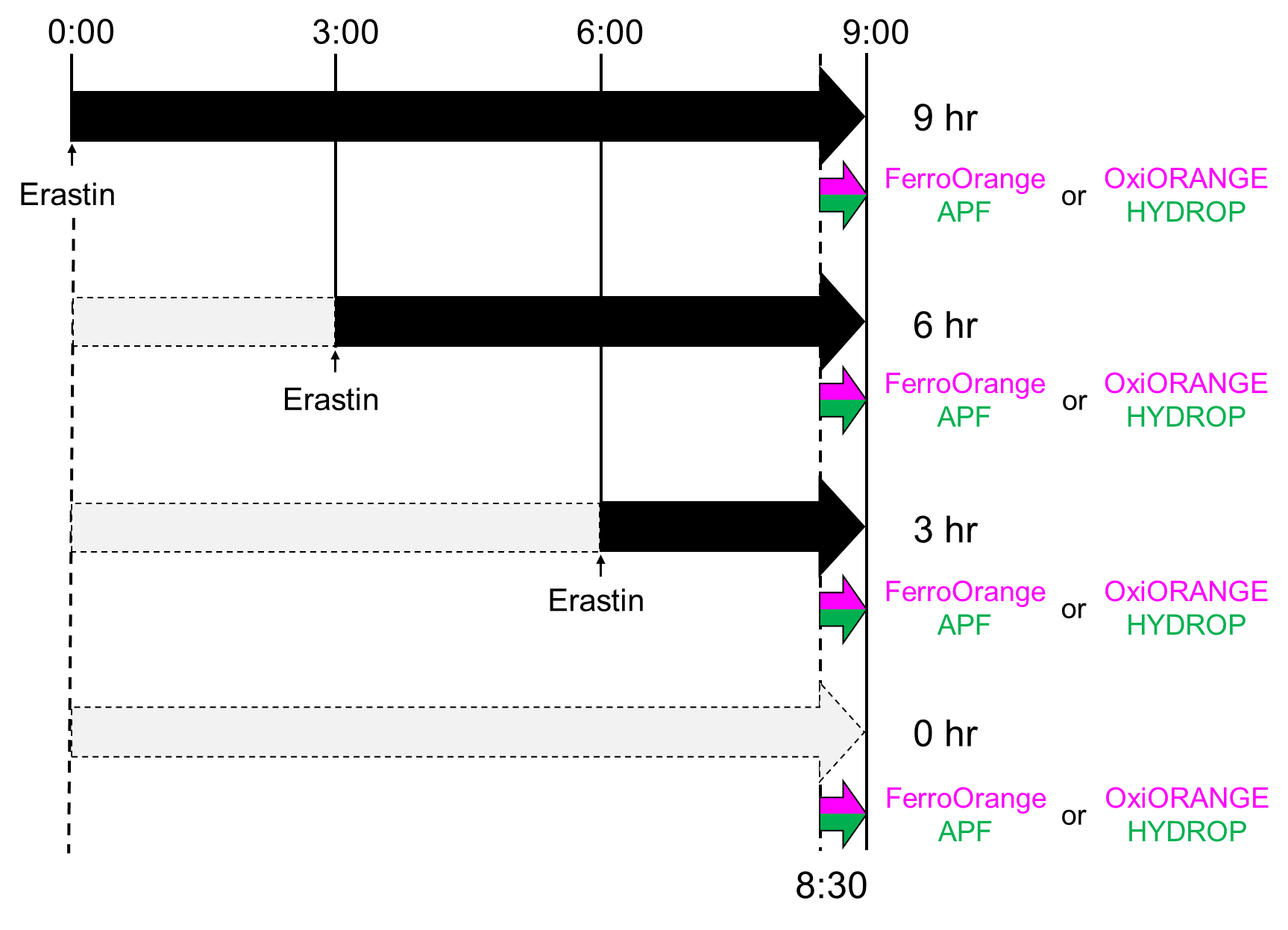
Actual timecourse of the experiment. Actually, erastin was added in different timepoints and addition of fluorescent probes and observations were performed simultaneously. Since these probes reacts with each target and fluoresces irreversibly, timecourse of ferroptosis was observed by the above procedure.